- The life cycle of stars and how they die
- Why more massive stars live shorter lives
- How the atoms that you consist of came to be
All material in the Universe is part of an infinite cycle, where old material is recycled and upgraded to become shiny new things, like stars. This means that all material we know of, even you and I, has once been part of a star. But how did we end up here? To answer that, we need not look at the evolution of ourselves, but rather the life and evolution of the stars.
In the beginning of the Universe, there was only hydrogen and helium, the very lightest of the elements in the periodic table (and a bit of lithium, but that is a story of its own). As such, the first generation of stars formed from cosmic gas clouds consisting more or less only of these two elements. All of the other heavier elements, like the oxygen we breathe and the carbon in our bodies, have been formed in various processes involving stars. But before we go into that, let’s take a closer look at how stars are formed.
The birth of a star
The space between stars is not always totally empty: gas clouds, the birth chamber of stars, can be found here. The gas clouds can start to cool, causing them to contract, creating clumpy, dense regions. These regions will accrete more and more, until they reach sufficient mass for the gas to begin collapsing, releasing its own gravitational energy in the process. The material at the centre begins to heat up as a result; as the density, temperature and pressure increase, the material will start to condense into a ball of super hot gas: a protostar is born!
Now, the protostar is not an actual star yet. For that, it needs to start fusing hydrogen into helium (this process is often referred to as burning, but it’s not entirely correct, as explained in a previous blog). To begin hydrogen fusion, the protostar needs to reach a core temperature of millions of degrees. For this, a large gravitational pressure is required, only achievable if the protostar has at least 8% of the Sun’s mass. If the hot ball of gas fails to gather that much mass, it will never start to fuse its hydrogen, and ends up as a brown dwarf [1].
Once the protostar depletes its surroundings from the infalling gas, which can take a few 100 000 years, it will have gathered enough mass to start fusion in its core. At the onset of fusion, the star leaves its protostar stage and (skipping any sort of child- and teenage phases) enters what will be a majority of its life—the main sequence .
Here it should be mentioned that many stars are born and found in pairs, known as binaries, and about one third of all stellar systems contain more than one star. Even though this story is about the life of single stars, it is worthwhile remembering that stars with companions can strongly influence each other’s evolution, where one of them might steal material from the other, causing its own evolution to speed up. But that, too, is a story for another day.
The middle ages
The name “main sequence” originates from the diagram that truly is astronomers' bread and butter: the Hertzprung-Russel diagram (or HR diagram, for short). This diagram shows the evolutionary stages of stars, and naturally we will be coming back to it a lot!
The HR diagram shows the relation between stars' colour (or temperature) and luminosity. There are only certain combinations of these properties that stars can have, which was discovered by Ejnar Hertzsprung and Henry Norris Russel in the early 20th century, and have given name to the diagram.
They noticed that a majority of the stars they observed end up on a diagonal sequence in the HR diagram, which they called the main sequence: the less massive, cooler and redder stars are found at the bottom, and the massive, hotter blues at the top (beware, the x-axis of the HR diagram is inverted!). To be quite frank, besides fusing hydrogen and trying to not collapse on itself (but that’s spoilers for what’s up ahead), the middle-aged main-sequence star doesn’t do much, and this is where most of the life of any star is being spent.
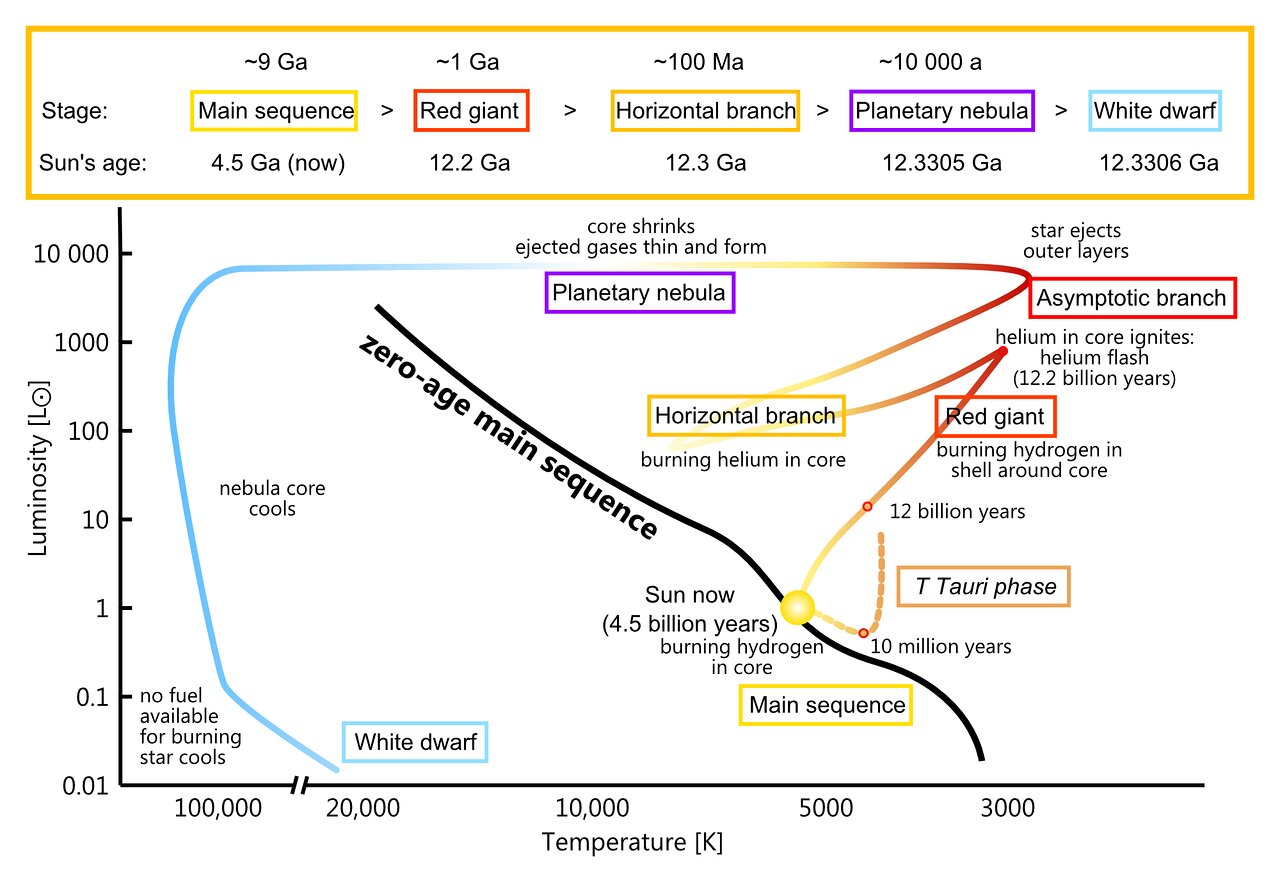
Despite what the name “sequence” suggests, stars don’t move along it, as their temperature and luminosity don’t change much at this stage. How long does this phase last? The more massive the star, the shorter it lives. This is explained by the fact that the higher the mass of the star, the higher its temperature is; the higher temperature speeds up the process of fusing hydrogen into helium, and the star will deplete its fuel faster. As an example, the most massive stars of more than 100 solar masses burn through their fuel in a few million years, whereas the ones with only a small fraction of the solar mass will live for tens of billions of years. How massive can a star be? Astronomers have found a star weighing more than 300 times the Sun's mass, a very rare and exciting star!
The life crisis
The life crisis of a star of low (~0.3 solar masses) to intermediate mass (8 solar masses), the perhaps-getting-a-motorcycle phase, hits when the hydrogen in the core is depleted and the star reaches a new phase in its life: the beginning of the end. When there is no more fusion of hydrogen providing outwards pressure, the core starts to collapse. To maintain in equilibrium, the outer parts, the envelope as astronomers call it, swell into a red giant (red supergiant in the case of very massive stars, between about 8 and 30 solar masses), and the star enters the red giant branch, seen on the top right on the HR diagram.
Whilst the envelope is expanding and cooling, in the core the compression increases both the pressure and temperature. The core will continue to compress until it reaches high enough temperatures for helium fusion. Helium will fuse to carbon, which can pair up with more helium to form both nitrogen and oxygen, key ingredients for life as we know it here on Earth.
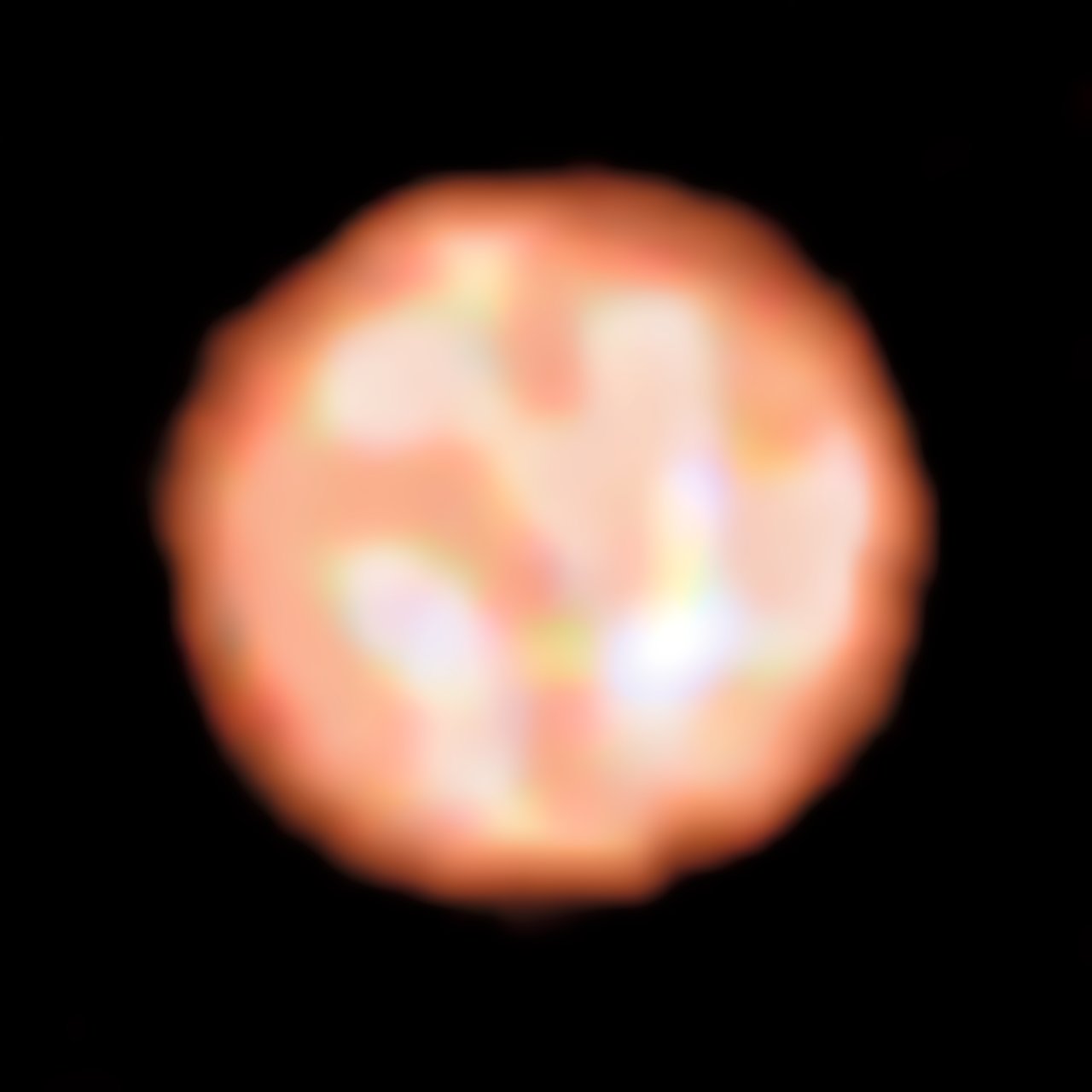
Dust from red giants actually includes some of the elements produced in their interior, like carbon. In a paper led by Julien Drevon of the Observatoire de la Côte d’Azur, France, astronomers used the MATISSE instrument on ESO’s VLT Interferometer to study the red giant star R Sculptoris and imaged carbon-rich dust in the making. True proof of humans being stardust!
Red supergiants are also often enshrouded by dust. Betelgeuse, the red star in the Orion constellation, is one of the closest red supergiants, only around 600 light years from Earth. In late 2019, the star suddenly became much fainter. A team led by Miguel Montargès at KU Leuven, Belgium, used the SPHERE instrument at ESO’s Very Large Telescope (VLT) to explain that the dimming of Betelgeuse was caused by the star casting out dust.
Post life-crisis
After the red giant branch and the initial life-crisis, the star has bought its motorcycle and is cruising down along the beach. But for the most massive stars, a bumpy ride awaits them.
When burning helium, the star moves from the red giant branch to the horizontal branch (named so because it is indeed horizontal in the HR diagram), and when burning carbon, it enters the second giant branch (which, due to historical reasons, is called the asymptotic giant branch). You might need to check the HR diagram above, because this can get confusing!
The names are of less importance, what matters is that the stars will move on the HR diagram, pulsating in brightness, with every new element it starts fusing. Because of this, the interior of stars ends up looking like an onion, with layers of progressively heavier elements the closer to the centre you get. But here the mass of the star is key again, since the more massive the star, the more massive the core, and the higher the capability to contract, meaning higher temperatures and –– yes, you guessed it –– fusion of heavier elements can take place!
Because of the difference in masses a clear distinction is made in how the stars’ lives eventually end.
The final stages
Stars don’t live forever. Stars with less than eight solar masses are not massive enough to fuse above oxygen (in rare cases up to neon and magnesium). Over time these stars leave their envelope, exposing the core: a white dwarf, supported by the pressure of tightly packed electrons. The remnant envelope can be lit up by the hot white dwarf, creating a planetary nebula (which has nothing to do with planets). Eventually the white dwarf will cool down, turning into a black dwarf.
The more massive stars will continue to create heavier and heavier elements in their core, compressing more and more and getting hotter and hotter. Until fusion reaches iron. Unlike fusion of lighter elements, which releases energy, fusing iron actually consumes energy. So once the core contains just iron, the outward pressure support of the star disappears, and it starts to collapse. For stars with masses around eight to 25 times the mass of the Sun, the intense gravity of the collapsing star crushes electrons and protons together to form neutrons, where the neutrons eventually will support the core, leaving behind an extremely dense neutron star.
For the most massive stars, the immense gravity will break the pressure of the neutrons and the core will collapse completely, forming a black hole. Paired with this collapse of material forming either a neutron star or a black hole, an explosion known as supernova often takes place. The conditions in these explosions are such that many elements heavier than iron can form.
However, we are not quite done yet. Remember the binaries from the beginning of this story? The ending lives of white dwarfs might not necessarily be too exciting. However, that doesn’t have to be the end: sometimes they too go out with a bang. If found in a binary with another star, the white dwarf can start stealing some of its companion’s material, causing the white dwarf to have a runaway fusion of material, resulting in a violent explosion that destroys the white dwarf in a supernova (or in the less violent cases, a micronova).
Additionally, if neutron stars are found in a binary with either a neutron star or a black hole, these can merge, creating some of the heaviest elements, such as gold.
We are all stardust
Through stellar winds, planetary nebula or supernova explosions, the newly created elements like carbon, oxygen, iron and gold are ejected into space, and will form new gas clouds. Eventually, those gas clouds will form new stars and the cycle begins again. This means that the next generation stars will have more of the heavier elements in them, and that stars carry a chemical fingerprint of their birth cloud, effectively acting as fossils of their birth environment.
Astronomers can measure these elements using spectroscopy, where the light from a star can be split up into its components, a spectrum, just like a rainbow. Every species of atoms leaves its own unique fingerprint in a star's spectrum, which can be captured with instruments such as ESO’s FLAMES and X-Shooter, among many others.
The story of stars and their life is not only an astronomical story, but also the story of physics, chemistry and about us, and how we came to end up on a planet around a rather ordinary star in a galaxy called the Milky Way.
Note
[1] While brown dwarfs aren’t massive enough to fuse conventional hydrogen, they can fuse deuterium, an isotope of hydrogen that consists of a proton and a neutron instead of just a proton. This can last for several million years before the brown dwarf runs out of deuterium.
Numbers in this article
- 300 solar masses is the most massive star found
- 8% of the Sun's mass to start fusion and become a star
- 8 solar masses: a star below this mass will end its life as a white dwarf
Links
Biography Rebecca Forsberg
Rebecca was a science communication intern at ESO. Prior to this position she completed a bachelors and masters degree in astronomy & astrophysics, and recently completed a PhD at Lund Observatory, Sweden. Rebecca found her passion for writing and communicating science as a science reporter for the Swedish magazines Populär Astronomi and Lundagård.